3D Magnetic Nanowire Networks for Neuromorphic Computing
Posted in News Story
A team of researchers led by postdoctoral fellow Dr. Dhritiman Bhattacharya, as part of the joint efforts between Prof. Kai Liu and Prof. Gen Yin’s groups in the Physics Department of Georgetown University, has demonstrated a novel material platform for implementing neuromorphic functionalities using 3-dimensional (3D) magnetic nanowire networks. Their results were recently published in ACS Applied Materials & Interfaces and presented by Dr. Bhattacharya in an invited talk at the 2025 American Physical Society Global Physics Summit in Anaheim, California.
In conventional artificial intelligence hardware, intelligence is encoded only in software. However, in the biological brain, intelligence emerges at multiple levels, starting from individual neurons. Neuromorphic computing aims to mimic this intelligence at the hardware level, with several emerging platforms leading the way. Among them, nanomagnetic devices have shown great promise due to their unique properties. Further enhancement of their multifunctional capabilities can be achieved by developing 3D magnetic nanostructures, but fabrication and characterization challenges have limited progress in this direction.
The researchers explored a novel 3D system consisting of interconnected networks of self-assembled magnetic nanowires (NWs), offering a simple and efficient fabrication process. These 3D magnetic NW networks closely resemble the self-organized architecture of the brain, where heterogeneous synaptic connections and complex interactions dictate functionality.
The networks were composed of Ni NWs, grown by Erin Marlowe. The networks were formed by sintering a collection of NWs and connecting them via electrodes. In these networks, the interconnected structure enables the formation of multiple unique transport pathways, determined by the presence or absence of NW intersections. Each pathway hosts discrete magnetization states that can be selectively addressed and electrically controlled using current pulses of varying magnitudes and durations. Additionally, the networks exhibit distinct features, such as history-dependent magnetic state switching and interconnected transport pathways.
Micromagnetic simulations were performed by Colin Langton and Dr. Zhijie Chen. Additionally, Langton, under the guidance of Prof. Gen Yin, performed simulations illustrating the potential of interconnected magnetic NW networks as reservoir layers in a neural network architecture, emphasizing their promise for neuromorphic computing. Other collaborators include Dr. Mahadi Rajib, Dr. Walid Al Misba and Prof. Jayasimha Atulasimha at the Virginia Commonwealth University, and Prof. Xixiang Zhang at the King Abdullah University of Science & Technology (KAUST), who carried out magnetic imaging studies and contributed to the understanding of the magnetic states of the networks.
These magnetic NW networks introduce an additional degree of freedom to control complexity and adaptability, as spin textures, magnetization dynamics, and NW coupling can be tuned not only by modifying NW geometry but also by leveraging the vast magnetic parameter space to design the system’s energy landscape. Efficient control of such a system holds great potential for low-power neuromorphic applications. Overall, this study lays the groundwork for future research into 3D multifunctional neuromorphic systems using this platform.
This work was supported in part by the National Science Foundation.
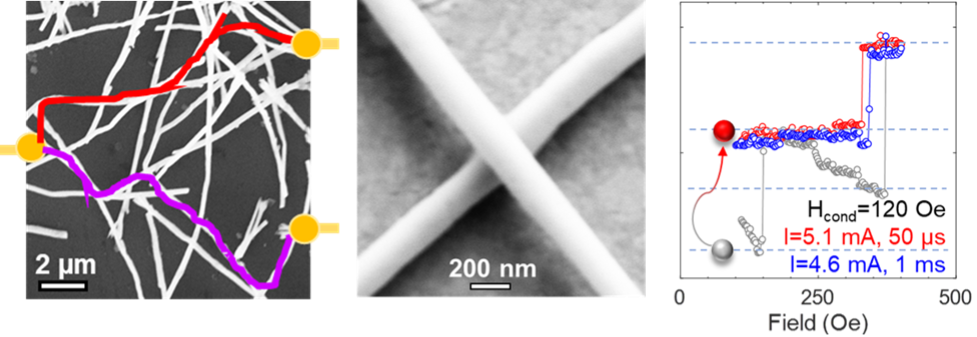